News Story
Energy Storage: Uncomfortable Numbers
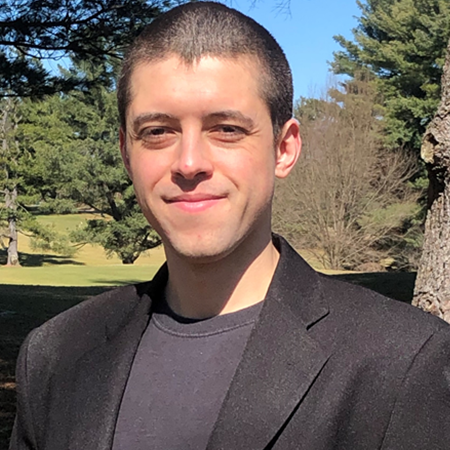
Electrochemical and thermal storage will be the pillars of decarbonization in the energy sector. There are some challenges with these technologies that are important to be aware of and addressed before it is perhaps too late. Electrochemical batteries (ECB’s), while indispensable components in modern society, need special attention: their energy densities are 40 times lower than fossil fuels, they have embedded 50-300 kg CO2,eq/kWh, costs range from $100-1000/kWh depending on the scale, and available Lithium (~14 million tons) will be depleted within the next century with the current mining rates, unless the ocean water Lithium become sustainably extractable, or revolutionary new technologies that no longer require critical materials arise. Thermal energy storage (TES), while they can be nearly twice as cheap and are more environmentally friendly, also have challenges: 2-3 times less energy dense than ECB’s, do not always offer energy savings and have more limited applications. While TES may have a relatively high value-proposition for hot water equipment in buildings, it can be challenging when integrating it with heat pumps; the additional space and weight required, coupled with a limited return on investment, become a hard sell to end-users when the benefits are not always tangible on a local level.
Introduction
The race for decarbonization is fueled by the current rate of growth of wealth and accelerated warming of our planet. Electrification seems to be the obvious path to enable a full integration of renewable energy into our energy matrix. With that, many challenges come along and it is paramount that we are aware and address them on the way, so we don’t shoot ourselves in the foot further down the road; we want to avoid another 1980’s heat pump fiasco - they are now being “re-discovered” four decades later. One such challenge is energy storage.
Storage is a fundamental piece of the puzzle in energy production, conversion, and use, since they allow for bridging the supply and demand at all times, improve resilience, and potentially efficiency, regardless of the source. What changes is the time scale and the storage medium.
In fossil fuels, the energy is stored in the fuels themselves naturally formed within millions of years, at the highest energy densities one can find (40-50MJ/kg or 11-14kWh/kg) - with exception of hydrogen (120MJ/kg or 33kWh/kg), and Uranium-235 (3.9TJ/kg or 1.1GWh/kg). Fossil fuels are also easily deployed to supply demand from the grid, albeit more inefficiently, but they serve as both energy (kWh) and power (kW) sources. Renewable energy from solar and wind are truly, and predominantly, power sources only, and highly intermittent, that’s why they will not be able to be directly integrated to the grid; it will lead to supply shortage or curtailment[i].
Electrochemical Storage
The road towards electrification will pose greater challenges in balancing the grid, hence we will have an increasing need for storage. Electrochemical batteries (ECB) are today the most mature technology; Li-Ion based batteries offer storage capacities of 200-300Wh/kg (0.2-0.3kWh/kg) which is 40 times less than fossil fuels. Even with the poor efficiency of heat engines (30-50%), storing fuels is still more convenient than storing electricity in batteries for the same output; this is especially relevant in aviation.
Furthermore, electrochemical batteries require important critical materials (e.g., Lithium and Cobalt) which we don’t have infinite sources of. In 2021, we mined 100 kilotons of Lithium, a 2.5 fold increase from 2016[ii]. We have on earth’s crust an estimate 14 million tons[iii], which would be enough for the next 140 years if current mining rate doesn’t increase, unless novel Lithium-rich sites are discovered, which is a possibility. Ocean water Lithium extraction might one day be a reality too[iv]. Additionally, today 60% of Cobalt is mined from the Democratic Republic of Congo, which draws a lot of concerns about human rights violations[v].
The processing of these materials also require high temperatures (800-1000°C) for which the heat source is primarily fossil fuels. Today 77% of the Li-Ion batteries are manufactured in China where coal is still the main primary energy sourcev. The embedded carbon in electrochemical batteries ranges between 50-300 kg CO2,eq / kWh[vi]. A Tesla Model 3, with an 80kWh battery has off-the-bat 2.4-16Tons of CO2,eq in it, which corresponds to 6,000-40,000 miles driven by an internal combustion engine car[vii].
Cost is also a tricky subject; for electric batteries the numbers range from $100-$1000/kWh, and it depends how they are computed, and on the scale of their application. For automotive industry, the current cost is $100-150/kWh[viii], and projected to be under $100/kWh in the next decade. For EV manufacturers the $50-100/kWh is the threshold range to make ECB’s economically competitive[ix]. At the utility-scale - which many may argue it will be the path forward for renewable energy integration - the current costs are in the range if $300-400/kWh[x],[xi] and projected to reduce to $100-250/kWh by 2050x. In residential scale the ECB costs can be in the range of $600-900/kWh[xii], making it very costly and perhaps economically infeasible for widespread deployment.
Prominent researchers and thinkers suggest that we always find an alternate, and possibly better, technology to solve roadblocks such as resource limitations[xiii],[xiv]. If that is true, then possibly in a few years Li-Ion battery challenges will (hopefully) no longer be a concern. Current non-Lithium based technologies such as the Vanadium Redox-Flow batteries, have shown some potential, but these still have an order of magnitude lower energy densities[xv], thus not yet effective competitors in large scale.
Thermal Storage
Thermal energy storage (TES) is a growing technology that will complement in the decarbonization race, but its applicability may be narrower than that of ECB’s. TES can be leveraged in buildings, where thermal loads range from 40-70% of total demand, and are the main drivers of peak loads[xvi]; TES can be a lower cost and possibly be more efficient solution in such applicationsxii. On the industry and power generation side, TES may have a room as well because it can serve for both heat and power demands. On the utility scale, however, the TES might have limited use, except perhaps on district heating, but not so much on grid balancing. For transport, TES may also be constrained due to packaging and weight limits.
The TES is more effectively done with latent thermal energy storage (LTES) where we store energy in Phase Change Materials (PCM’s) such as paraffin waxes (organic) and salt hydrates (inorganic), which are far more environmentally friendly and cheaper compared to the abovementioned critical materials. The storage densities, however, are in the range of 50-200Wh/kg which is 2-3 times less than ECB’s.
PCM’s cost can range from $1-100/kWh[xvii] and require much less-intensive production processes which will result in considerably lower carbon footprint as well, however the actual embedded carbon values need further investigation. Current commercial thermal batteries for hot water applications are in the range of $200-600/kWht[xviii], approximately half the price of a residential ECB, although installation costs may not be included.
TES has big challenges that need answers as well. First, like ECB’s, TES needs to be recharged, so unless it can be done with renewables directly (e.g., concentrated solar), it will require either fossil fuels or heat pumps. While TES can be effective in load shifting, it does not always result in overall energy savings. Second, the peak shaving, will result in cost savings, but with relative low electricity prices (US average $0.1/kWh[xix]), the return on investment (ROI) is not always attractive unless there are considerable subsidies. Furthermore, the impacts of TES are not always tangible to the end-user experience, if their thermal demands are met either way. This is particularly challenging when integrating TES with heat pumps for space heating and cooling, where, for instance, a storage of 80kWh capacity will result in an additional large box with the weight of a small car; is a hard sell to building owners. The highest value-proposition from TES in buildings is probably hot water, where the TES can replace the water tank entirely, providing overall size and cost savings.
Alternate Storage Technologies
There are many other energy storage technologies that fall in different regions of the power vs energy density trade-off map; some with specific niche markets such as pumped hydro, compressed air and flywheels[xx]. Many inadvertently classify fuel-cells as storage devices, but the energy is not stored in the cell, but in the fuel, i.e., Hydrogen. The latter, however, has its own realm of challenges and will be the topic for another editorial.
[i] Energy Storage Requirements for Achieving 50% Solar Photovoltaic Energy Penetration in California (nrel.gov)
[vi] CO2 Footprint and Life‐Cycle Costs of Electrochemical Energy Storage for Stationary Grid Applications (wiley.com)
[ix] Tesla 4680 cells, $60/kwh target, fuel-cell trash trucks, Ford batteries: Today’s Car News (greencarreports.com)
[xiii] Harari, Yuval N. author. Sapiens : a Brief History of Humankind. New York :Harper, 2015.
[xiv] Vaclav Smil, How the World Really Works, Viking, 1st ed. 2022
[xv] A Stable Vanadium Redox‐Flow Battery with High Energy Density for Large‐Scale Energy Storage - Li - 2011 - Advanced Energy Materials - Wiley Online Library
Published October 6, 2022