News Story
Layer by Layer: CEEE Builds Reputation in 3D Printing of HVAC Components
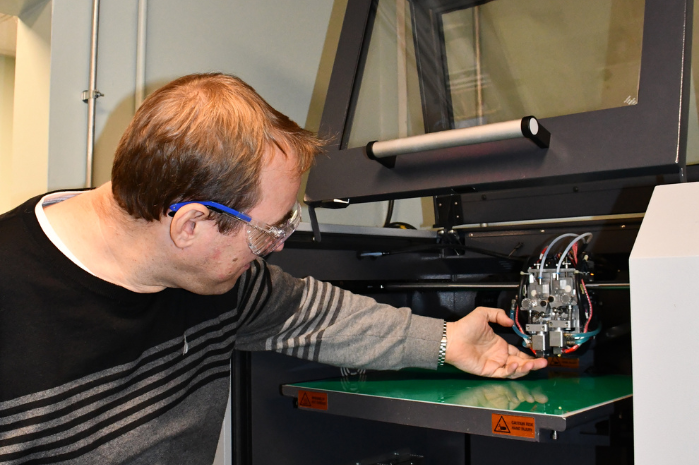
Lab manager Jan Muehlbauer adjusts a 3D printer in CEEE's Daikin Energy Innovation Laboratory.
The whirring of a 3D printer as the printhead moves back and forth rapidly, adding layer after layer to a design, has become a familiar sound in the labs where Center for Environmental Energy Engineering (CEEE) researchers work. In the past decade, additive manufacturing, colloquially known as 3D printing, has become a powerful tool for designing the components used for heating, ventilation and air conditioning (HVAC), and has opened up the ability to incorporate different materials and novel structures that improve efficiency, enhance sustainability and lower costs. Here are four CEEE projects taking advantage of the power of additive manufacturing:
Cool data
In CEEE’s Small and Smart Thermal Systems Laboratory (S2TS), spools of polymer filament and metal wire are fed into a 3D printer and transformed into components that could help cool the expanding number of data centers that keep our data-rich society afloat. Data center cooling is energy intensive, often accounting for 33-40% of the overall data center energy usage, and consumes hundreds of billions of gallons of fresh water each year.
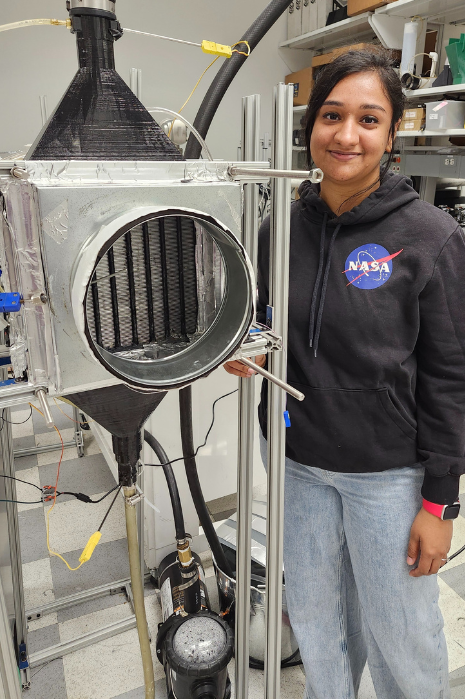
Through the U.S. Department of Energy (DOE) Advanced Research Projects Agency-Energy’s COOLERCHIPS program, CEEE and its partners are developing a compact, high-performance, energy-efficient, sustainable solution for high-efficiency air cooling of edge data centers that uses non-water-based cooling. For CEEE’s portion of the project, researchers are designing a cost-effective additive manufacturing-enabled dry cooling technology. Only less than 5% of the power fed to the data center can be used for cooling purposes – this will be a record-high air-cooling efficiency.
Using an additive manufacturing technique called Embedded Fiber Composite Additive Manufacturing, which utilizes a modified Fused Filament Fabrication (FFF) process, the printer operates with two print heads. One head deposits the polymer to form the channel walls, while the other embeds metallic wires into the polymer structure as it is being built. The final result is a heat exchanger with metallic fins that has microchannels made out of polymer materials. The arrangement, referred to as a cross-media heat exchanger, provides direct heat exchange between the hot and cold fluids with the help of protruding fins and without any leakage – an effect that would be nearly impossible to achieve without a 3D printer.
“We are embedding the metal in the plastic to create a heat exchanger that is low cost, compact and can dissipate high amounts of heat,” explains researcher Shruti Menon. The project's principal investigator (PI) and co-inventor is CEEE Co-Founder Michael Ohadi, and the co-PIs are Research Professor Amir Shooshtari and Research Assistant Professor Andres Sarmiento.
While the 3D layering technique is central to the design, it also poses challenges. “The problem with 3D printing is that there are small gaps between the layers, which can be potential leak points if not designed properly,” says Menon. The team’s current goal is to demonstrate the technology on a ~2.5 kW system, with an eventual objective of scaling up to an efficient and effective solution that could cool 1.5 MW, and slash data-center energy usage.
A high-pressure situation
While Menon’s research team focuses on cooling the metaphorical “cloud,” some of her S2TS colleagues are leveraging additive manufacturing to design components to cool the turbines on the aircraft flying among the real clouds. Through a DOE-sponsored project, the research team – which includes graduate research assistant Zhengda Yao and Mechanical Engineering Professors Amir Shooshtari, Hugh Bruck and Michael Ohadi – is developing extreme environment heat exchangers for use in aerospace, transportation, electricity generation, power plants, waste heat recovery and more. The project targets heat exchangers capable of operating in temperatures and pressures exceeding 800 degrees Celsius and 80 bar, respectively.
Plastic can’t withstand such high temperatures and high pressure, so metal is the material of choice. The research team turns to the A. James Clark School of Engineering’s Terrapin Works for help. Terrapin Works is home to a vast array of industrial 3D printers, including a ProX200 machine designed to manufacture intricate metal parts through a process called direct metal laser sintering. Metal powder – composed of nickel-based Inconel superalloys – is melted, fused together at the molecular layer with a laser beam, and deposited layer-by-layer to fabricate compact microchannel heat exchangers. Inconel superalloys offer exceptional strength and corrosion resistance, making them ideal for high-temperature, high-pressure environments.
“This technique allows us to make features that are very small,” Yao explains. “Our microchannels are smaller than 700 microns. That means we can fit more microchannels, which improves the heat transfer.” To test the technology’s thermal-hydraulic performance, Yao heads back to the S2TS lab, where he’s constructed a high-pressure test loop using supercritical CO2 as the cooling medium.
Testing indicates that the compact heat exchanger prototype can bring a higher power density than the current state of the art. “It’s smaller and offers higher performance and efficiency,” Yao says. “You’re going to use smaller space and less fuel,” he says, “and that means lower costs.”
Next-generation heat exchangers
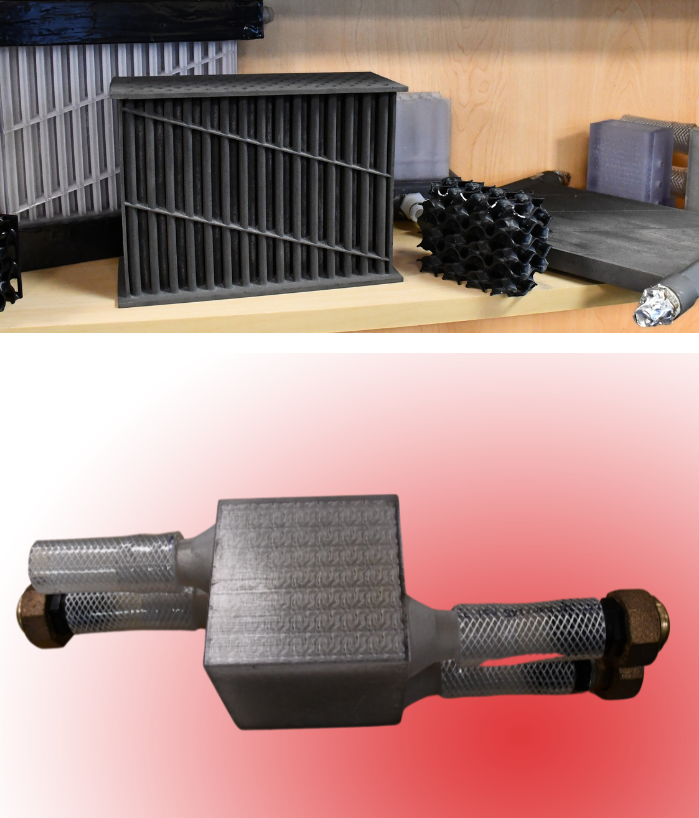
The 3D-printed prototypes that line the shelves of CEEE’s Daikin Energy Innovation Laboratory showcase the last decade’s heat exchanger innovations – an important stage in the center’s 30-plus years of HVAC breakthroughs. There’s the titanium heat exchanger that CEEE fabricated in 2016 through direct metal printing, with the help of partner 3D Systems and support from DOE’s Building Technologies Office. The prototype weighs 20% less and has 20% less volume than traditionally manufactured heat exchangers.
There’s a polymer heat exchanger featuring an array of tubes, each shaped differently. “Every single tube is customized to a shape that will create the best heat transfer with the least pressure drop,” explains Senior Faculty Specialist Jan Muehlbauer, who oversees the Daikin Lab. “The nice part about 3D printing is that it gives you so much flexibility to prototype different designs. If you wanted to build a prototype with conventional technologies and extrude tubes and feed them together, it would be so complicated and expensive.” He notes that 3D printing is frequently used for rapid prototyping of HVAC components, but once the design is finalized, more traditional manufacturing techniques are typically more cost-effective for mass production.
Today, CEEE researchers continue their quest for next-generation heat exchangers. Some of their newest prototypes utilize Triply Periodic Minimal Surfaces (TPMS), complex, compact undulating designs that are gaining traction in many engineering applications, including heat exchangers. This is mainly driven by advancements in additive manufacturing that enable reliable TPMS-based prototypes for the first time. These surfaces are of particular interest to the heat transfer community for a multitude of reasons. First, they are highly compact. A large heat transfer area is packed into a given volume, which allows for smaller heat exchangers. They also have a high mechanical performance, which means that the smooth and self-supporting structure enables high pressure fluids such as supercritical CO2 with small wall thicknesses. In addition, they have a high heat transfer, which originates from the complex, and intertwined flows in very small channels. And, due to their continuous, repeating, and smooth surface, these types of heat exchangers have a low pressure drop, which means they require less pumping power and are more efficient.
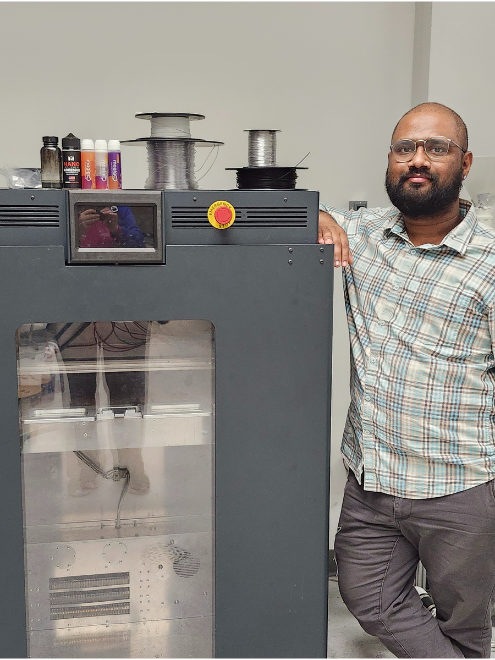
When the sun sets on a cold winter day and the temperature plummets, it follows that a heat pump consumes more energy to keep a building at a comfortable temperature. What if you could store the warm thermal energy from the daytime? That’s the idea behind thermal energy storage (TES), which can be used to optimize heat pump efficiency and control costs through peak load shifting. TES allows building owners to store energy when it’s least costly and use it during more expensive peak periods. The same idea can be applied in the summer, with the cooler nighttime thermal energy stored for daytime use.
While the idea is simple in theory, engineers have struggled to innovate cost-effective TES systems with high power density. Many TES systems are available in the marketplace, but compact ones are scarce. Using the FFF additive manufacturing technique, a CEEE team is innovating a low-cost, compact TES. The prototype is a cross-media construction of polymer embedded with thermal conducting aluminum wires that is expected to yield a 10-50% improvement in power density over state-of-the-art heat exchanger geometries. The work is supported by DOE’s Buildings Energy Efficiency Frontiers and Innovation Technologies (BENEFIT) program. Mechanical engineering professors Michael Ohadi and Amir Shooshtari are the UMD principal investigators on this project.
Researcher Vereesh Ayyagari envisions that the technology will be commercialized eventually and used to supplement heat pumps. “With this technology, you won’t need to buy as big of an air-conditioning unit,” says Ayyagari, a fourth-year doctoral student, “because the TES will give you a boost whenever your AC is running at its peak. So you can reduce your equipment costs and your energy costs, all while lowering emissions.”
Published December 3, 2024